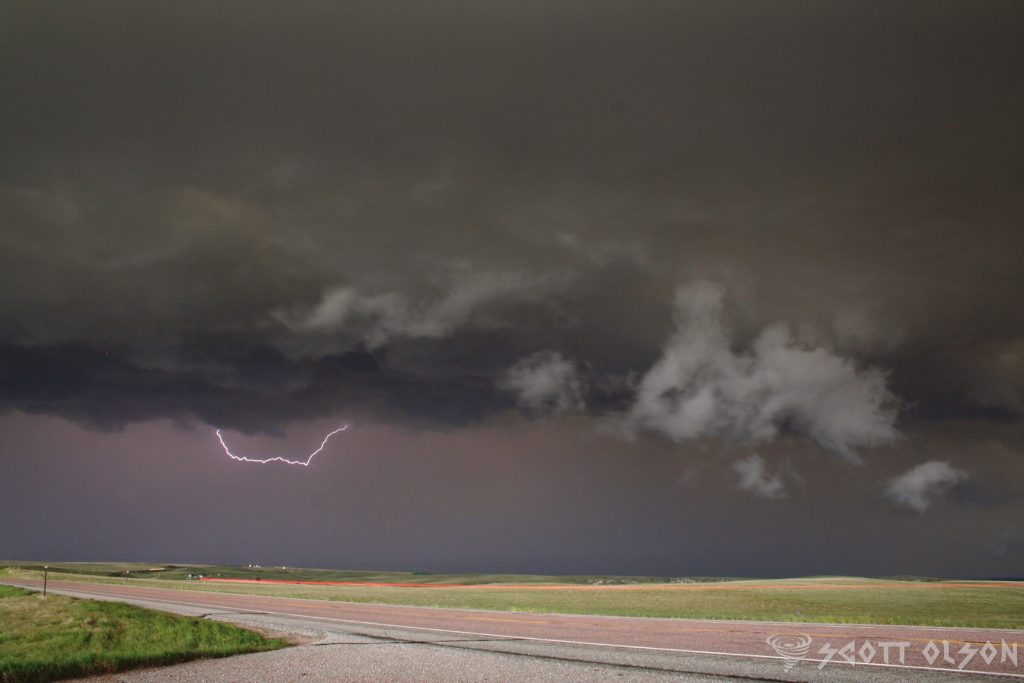
Storms are among the most powerful and awe-inspiring natural phenomena on Earth, capable of producing heavy rain, strong winds, large hail, and even tornadoes. But what causes these storms to form, and what factors influence their severity? The answer lies in the various types of atmospheric boundaries that exist in our atmosphere, including drylines, warm fronts, cold fronts, and outflow boundaries.
Drylines are a unique type of boundary that is most commonly found in the Great Plains region of the United States. A dryline separates warm, moist air from hot, dry air and is often characterized by a sharp contrast in temperature and humidity across the boundary. As the sun heats the ground during the day, the hot, dry air on one side of the dryline rises, creating a localized area of low pressure. This low-pressure area then draws in the warm, moist air from the other side of the boundary, setting the stage for severe thunderstorm development.
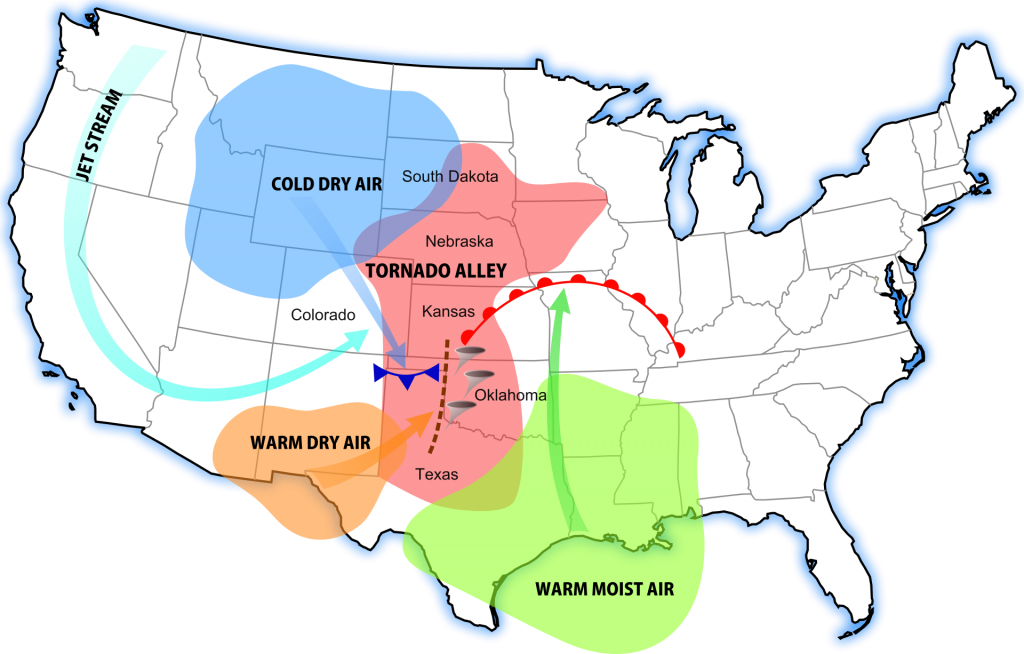
Dryline storms are notorious for their rapid development and intense nature. As the warm, moist air is forced upward by the rising hot, dry air, it cools and condenses, forming towering cumulus clouds that can quickly evolve into severe thunderstorms. These storms often produce large hail, damaging winds, and even tornadoes, making them a significant threat to life and property in the regions where they occur.
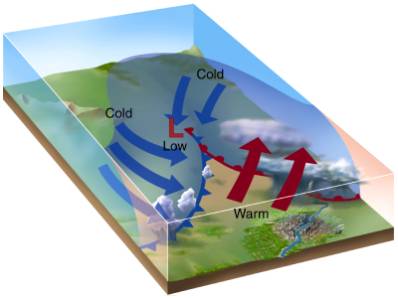
Warm fronts, on the other hand, are a type of boundary that occurs when a mass of warm air advances into a region of cooler air. As the warm air rises over the cooler air, it cools and condenses, forming clouds and precipitation. Warm front storms are generally characterized by widespread cloudiness, steady rain, and occasionally, thunderstorms.
One of the unique features of warm front storms is the gradual nature of their development. Unlike dryline storms, which can form and intensify rapidly, warm front storms tend to develop slowly over a period of several hours or even days. This slow development can lead to prolonged periods of rain and cloudiness, which can have significant impacts on local weather patterns and human activities.
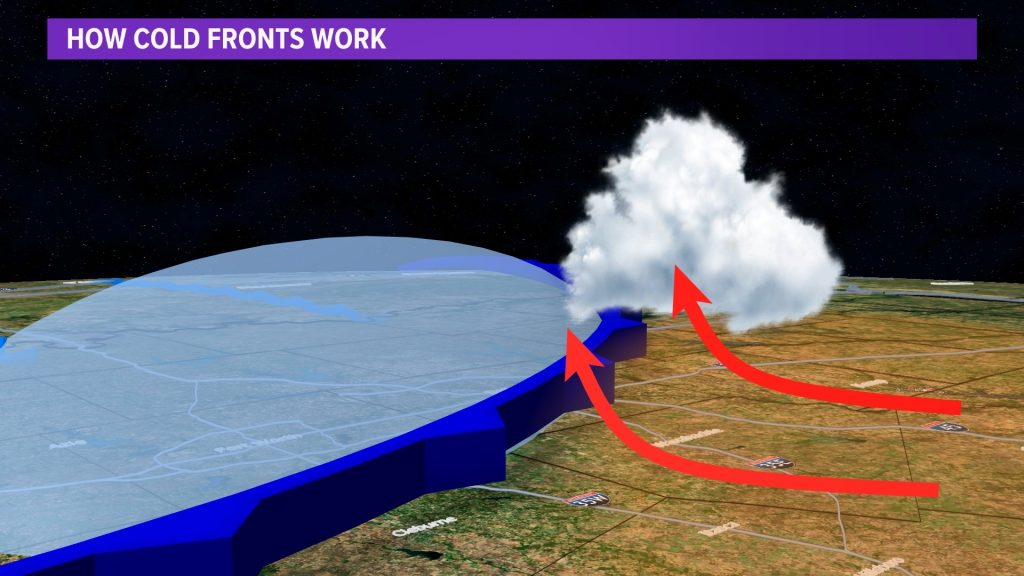
Cold fronts are perhaps the most well-known type of atmospheric boundary, and for a good reason. These boundaries occur when a mass of cold air overtakes a region of warmer air, forcing the warm air to rise rapidly. As the warm air rises, it cools and condenses, forming a line of intense thunderstorms known as a squall line.
Cold front storms are often accompanied by a dramatic change in weather conditions, with temperatures dropping sharply and winds shifting direction as the front passes through. These storms can produce a wide range of severe weather hazards, including damaging winds, large hail, and tornadoes, making them a significant threat to life and property.
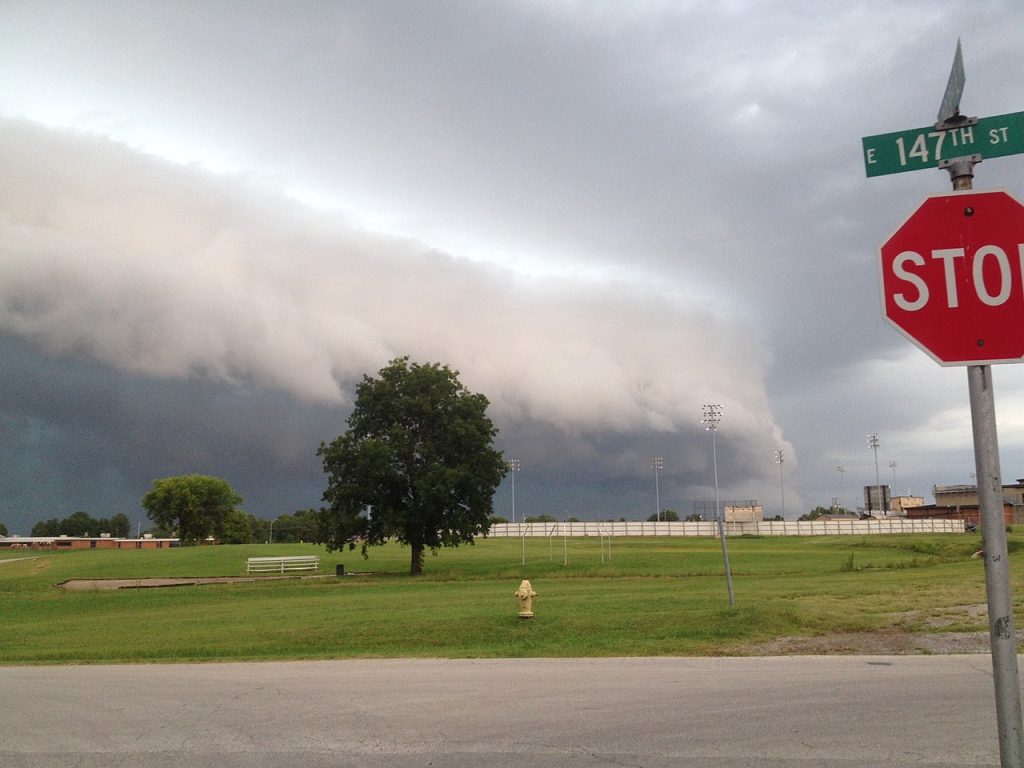
Outflow boundaries are a type of boundary that forms when cool, dense air from a previous storm or rain-cooled air rushes out ahead of the storm, creating a mini cold front. As this cool air undercuts the warm, moist air ahead of it, it can trigger the development of new thunderstorms, often in a repeating cycle known as “training.”
Outflow boundary storms can be particularly dangerous because they can form quickly and with little warning, catching even the most prepared individuals off guard. These storms often produce heavy rain, strong winds, and occasionally, severe weather hazards such as large hail and tornadoes.
While atmospheric boundaries are a critical factor in the formation of severe storms, they are not the only factors at play. Moisture, instability, and wind shear are also essential ingredients in the development of severe thunderstorms and tornadoes. When these factors combine with a strong atmospheric boundary, the result can be a significant severe weather outbreak.
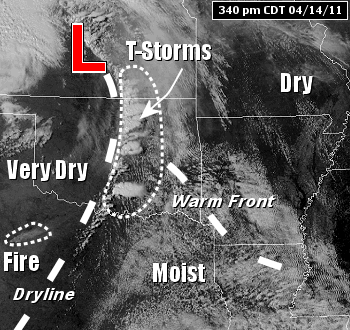
Drylines and Tornadoes: Drylines are known to be a significant factor in the formation of severe thunderstorms and tornadoes, particularly in the Great Plains region of the United States. A study by Hoch and Markowski (2005) found that “70% of all tornadoes in the southern Great Plains from 1999 to 2001 occurred within 100 km of a dryline.”
One notable example of a dryline-related tornado outbreak occurred on May 3, 1999, in Oklahoma and Kansas. According to the National Weather Service, this outbreak produced 74 tornadoes, including the devastating F5 tornado that struck Moore, Oklahoma, causing 36 fatalities and over $1 billion in damage.
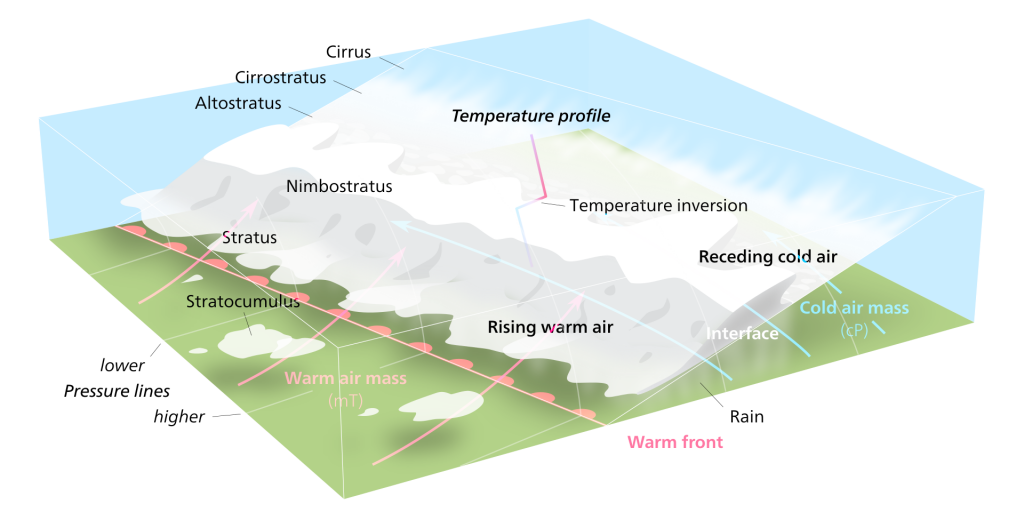
Warm Fronts and Tornadoes: While warm fronts are less commonly associated with tornado formation than other boundary types, they can still contribute to the development of severe thunderstorms and occasional tornadoes. A study by Gaffin and Parker (2006) found that “approximately 10% of all tornadoes in the United States are associated with warm fronts.”
An example of a warm front-related tornado event occurred on March 2, 2012, in the Ohio Valley region. The National Weather Service reported that an EF4 tornado touched down in Henryville, Indiana, causing significant damage and 11 fatalities. This tornado was associated with a warm front that had been draped across the region, providing the necessary moisture and instability for severe storm development.
Cold Fronts and Tornadoes: Cold fronts are a well-known contributor to severe thunderstorm and tornado formation. A study by Trapp et al. (2005) found that “approximately 60% of all tornadoes in the United States are associated with cold fronts.”
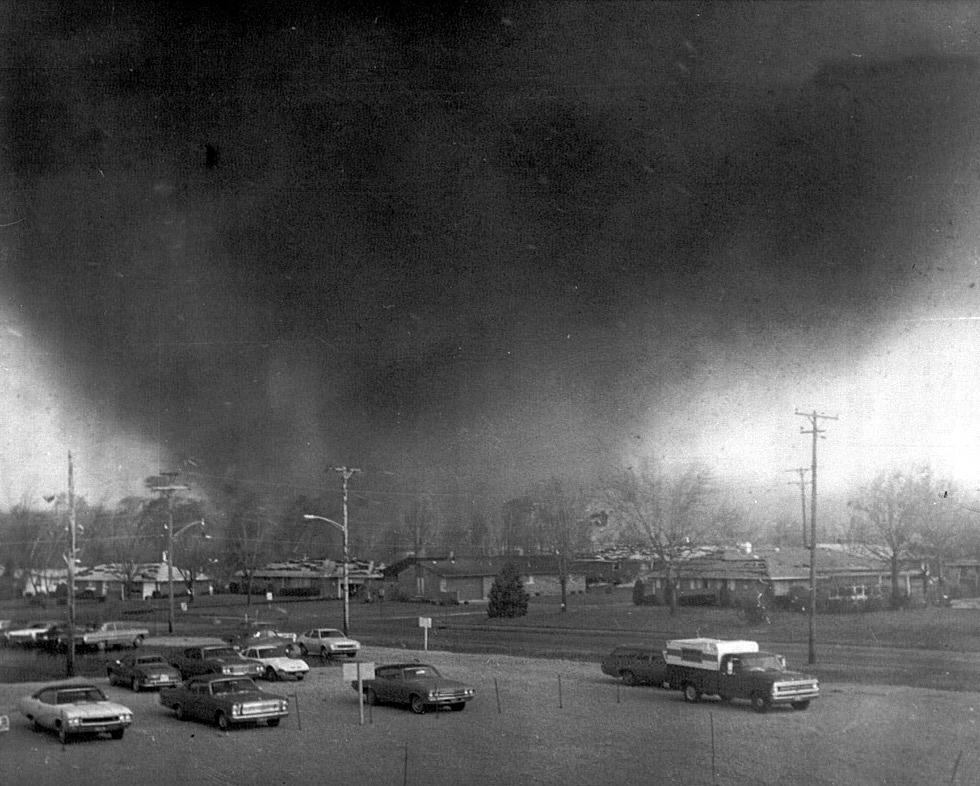
The “Super Outbreak” of April 3-4, 1974, is a prime example of a cold front-related tornado event. During this outbreak, 148 tornadoes touched down across 13 states, causing 330 fatalities and over $600 million in damage (adjusted for inflation). The outbreak was associated with a powerful cold front that swept across the eastern United States, providing the necessary ingredients for widespread severe weather.
Outflow Boundaries and Tornadoes: Outflow boundaries, which are created by the cool, dense air that flows out of thunderstorms, can also contribute to tornado formation. A study by Markowski et al. (1998) found that “nearly 20% of all tornadoes in the central United States are associated with outflow boundaries.”
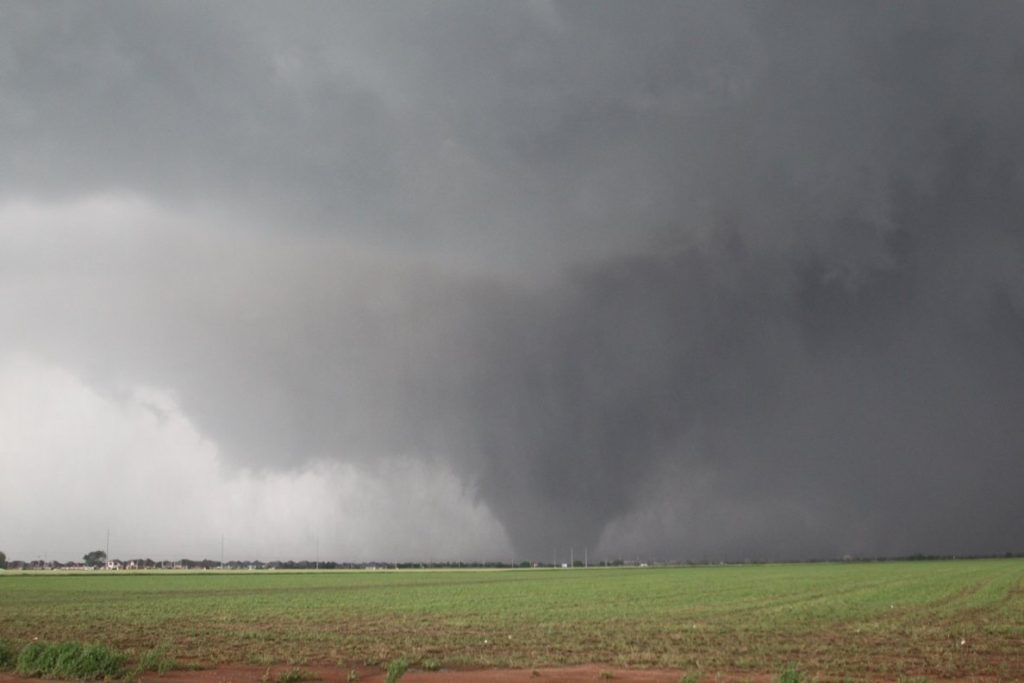
One example of an outflow boundary-related tornado event occurred on May 20, 2013, in Moore, Oklahoma. On this day, an EF5 tornado touched down, causing 24 fatalities and over $2 billion in damage. The tornado formed along an outflow boundary that had been generated by earlier thunderstorms in the area, highlighting the importance of these boundaries in severe weather formation.